Seashell Architecture
Marine snails don’t find their shells, they make them. As they grow, they secrete calcium carbonate to build protective exoskeletons that scale with their bodies. These shells serve as both armor and housing, evolving into highly specialized forms that reflect each species’ movement, feeding strategy, and environment. While the exterior of a shell hints at its purpose, the internal architecture—how the structure actually works—is sealed off from view.
Industrial CT scanning changes that. Through scans, we can inspect these delicate structures non-destructively, in full 3D, and at resolutions fine enough to reveal growth patterns, material distribution, and the spatial logic of forms evolved over millions of years. The scans below examine six different shell types, reflecting distinct structural solutions to the same basic challenge: how to live and grow inside a mineral shell.
Moon Snail Shell
Let’s start with the most familiar form in this collection. The shark eye, a type of moon snail, builds a smooth, rounded shell with a low spire and wide, domed base. It’s not built for intimidation or ornament—it’s built for movement. These snails live right beneath the sand’s surface, gliding through sediment as they hunt buried clams. Their shell minimizes drag, hides them from view, and offers uniform protection without excess weight.
In the orbiting video visualization, we can see how the internal structure supports that function. The coil is wide and shallow, wrapping around itself in a low spiral rather than rising upward. There are no internal partitions or braces, just continuous curvature that distributes pressure evenly across the surface. The entire shell acts like a dome under compression, built to resist force from every angle. It’s a structure that feels less like armor and more like infrastructure: quiet, efficient, and remarkably effective.
Open Moon Snail Shell
Turrid Shell
This slender shell belongs to a turrid, a family of marine predators known for speed and efficiency in catching prey. Its tall spire, narrow profile, and fine axial ridges give it a streamlined shape ideal for life in shifting sands and gravel, where maneuverability is more valuable than bulk. Unlike more heavily armored species, the turrid doesn’t rely on external defenses. It relies on being fast, light, and difficult to catch.
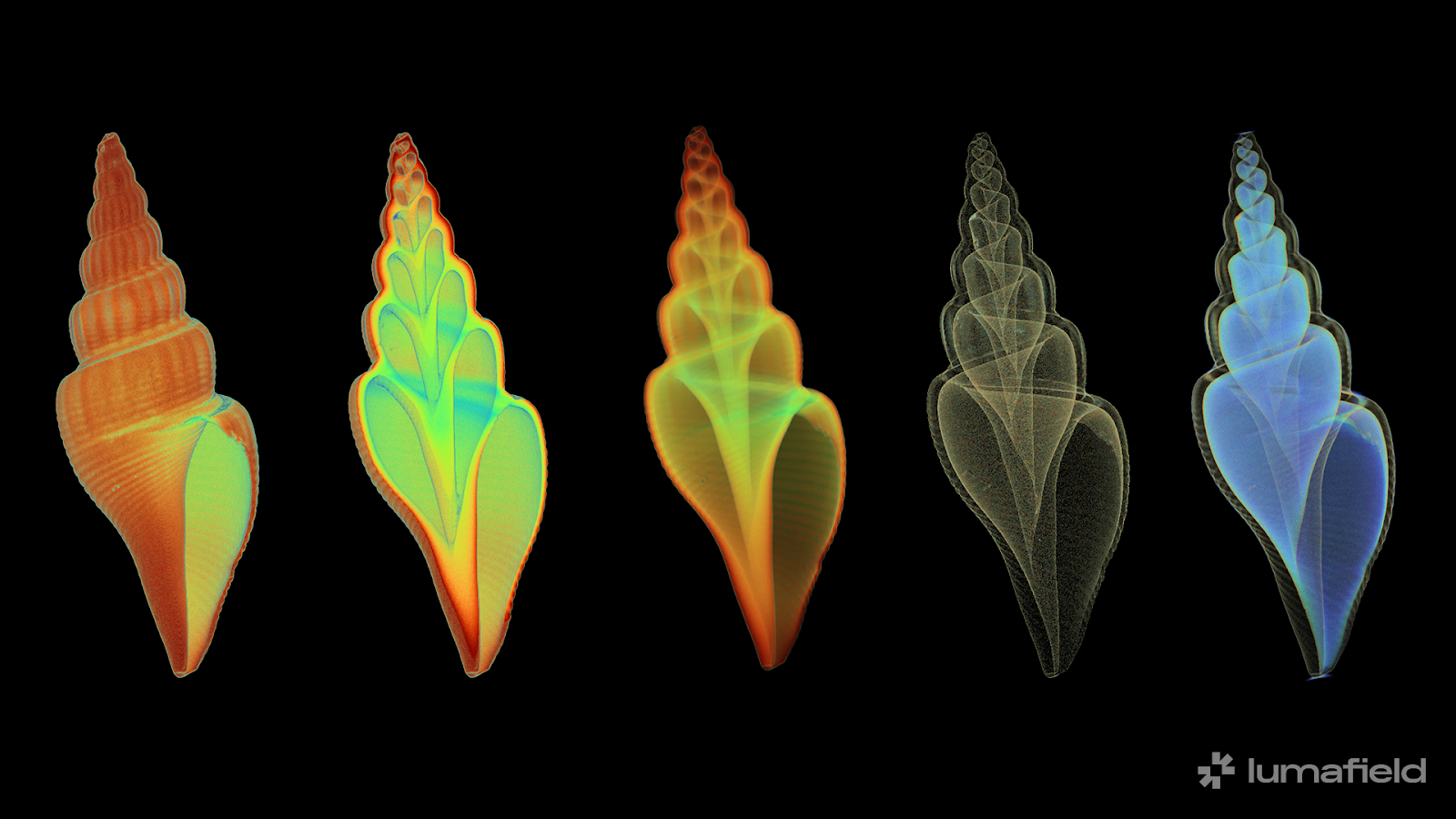
Internally, CT scanning reveals a tight, uninterrupted spiral with no sealed chambers or reinforcing struts. The structure is elegant in its simplicity: a continuous coil that maximizes internal volume while minimizing material use. Density mapping shows that the shell’s mass is concentrated in the apex, where the earliest growth formed a solid core, and the outermost wall, which serves as the first line of defense. Everything else is open, hollow, and shaped for efficiency. It’s a shell that isn’t trying to be strong everywhere—only where it counts.
Open Turrid Scan
Cone Shell
At first glance, a cone shell appears almost mathematical: clean, conical, and unornamented, with a polished surface that conceals its deadly role. Members of the Conidae family, including this specimen, are specialized hunters that deliver venom through a harpoon-like radula. The shell’s streamlined shape supports that behavior. It is dense, smooth, and built to move easily through water or sand with minimal resistance.
CT reveals an interior that echoes the simplicity of the exterior but adds an unexpected structural logic. Here, outer walls are considerably thicker than those inside, which are paper thin and delicately bound to a central columella that tapers dramatically from bottom to top. In cross-section, these braces form a tree-like pattern that distributes force efficiently across the shell’s body. This internal architecture is subtle, lightweight, and remarkably strong.
Open Cone Scan
Turritella Shell
The Turritella shell exemplifies structural repetition: each whorl builds directly on the last in a perfect logarithmic progression. Its spiral isn’t just tall, it’s architectural, rising in stacked increments like a helical column. From the outside, it looks regular; inside, it’s even more precise.
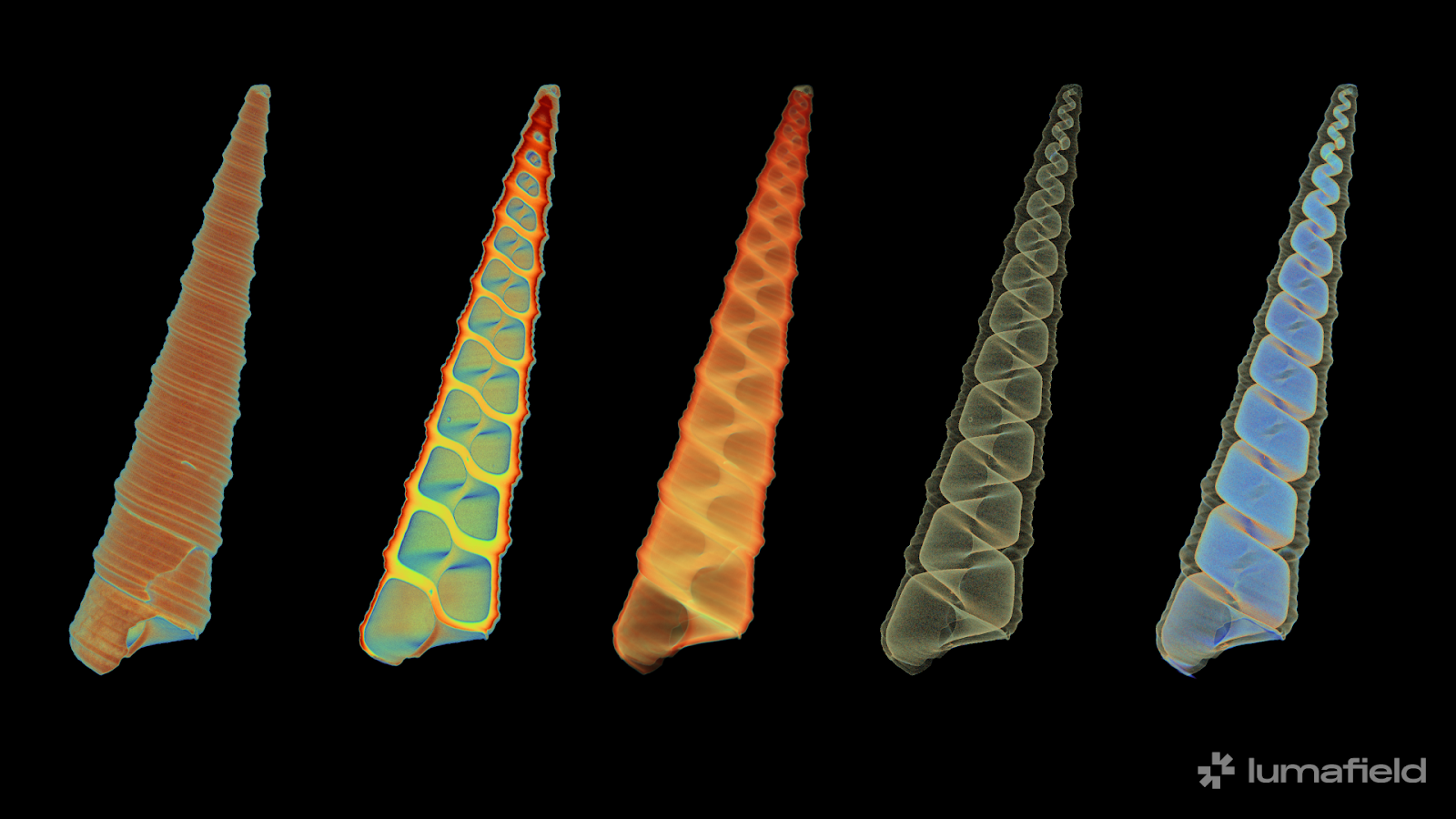
CT shows how growth is recorded in each successive twist of the helix. Older whorls taper toward the apex, culminating in a dense point. Unlike the other shells in this group, the turritella lacks a thick central columella. Instead it’s more of a pure corkscrew relying more on the external wall for its strength across growth cycles.
Open Turritella Scan
Murex Shell
The branched murex shell is more fortress than home. Heavy spines erupt from thick ridges, each one a defensive structure warding off predators. But seen in the edge-view orbital video, CT scanning strips away the drama of the exterior and reveals the system underneath. The spines are hollow, saving weight without sacrificing reach. And more importantly, the interior tells us how the snail used this shell.
At the core is the columella—the spiral’s load-bearing axis—reinforced with a series of pronounced internal ridges. These aren’t merely structural; they’re functional for the animal. As the snail grows, it grips the columella with its body, anchoring itself against the torque of movement or external force. The ridges give it traction, like a screw thread or ladder rung, allowing it to retract deeper into the shell and brace itself. Without CT, these practical features are invisible. With it, we see both how the shell was made, and how it was used by the snail living inside.
Open Murex Scan
Conch Shell
Even in its early stages, this horse conch displays the makings of a large-scale structure. The spire is tall and flared, and axial ridges add stiffness and surface complexity. Unlike the Turritella, the growth pattern here is less uniform and more adapted to scale. It’s a blueprint for helping the largest horse conches grow up to two feet in length without losing mechanical efficiency.
Use the embedded Voyager viewer below to move the revolving slice slider and explore cross-sections of the conch shell anchored to its central columella—revealing how internal structures spiral and shift with each degree of rotation.
The conch’s curved partitions follow the spiral without sealing off earlier chambers, adding reinforcement without taking on too much material. We’re able to trace these supports from base to tip, giving a clear picture of how a conch manages the tradeoff between size and strength while keeping the shell light enough to move.
Open Conch Scan
Quantifying Shell Strength
CT not only opens up the internal world of a shell; it lets us measure it. With Voyager’s Wall Thickness Analysis, we can map exactly how material is distributed and how that distribution reflects the stresses of life. In this turrid shell (below, top left), we see a familiar pattern: the outermost lip of the aperture is the thinnest region, highlighted in blue. That’s because it’s the newest part of the shell, freshly grown and not yet reinforced. In contrast, the columella (the central pillar) is the thickest area. For the turritella shell (below, bottom left), the thickest points of the shell are the joints on the outer wall between one rung of the spiral and the next.
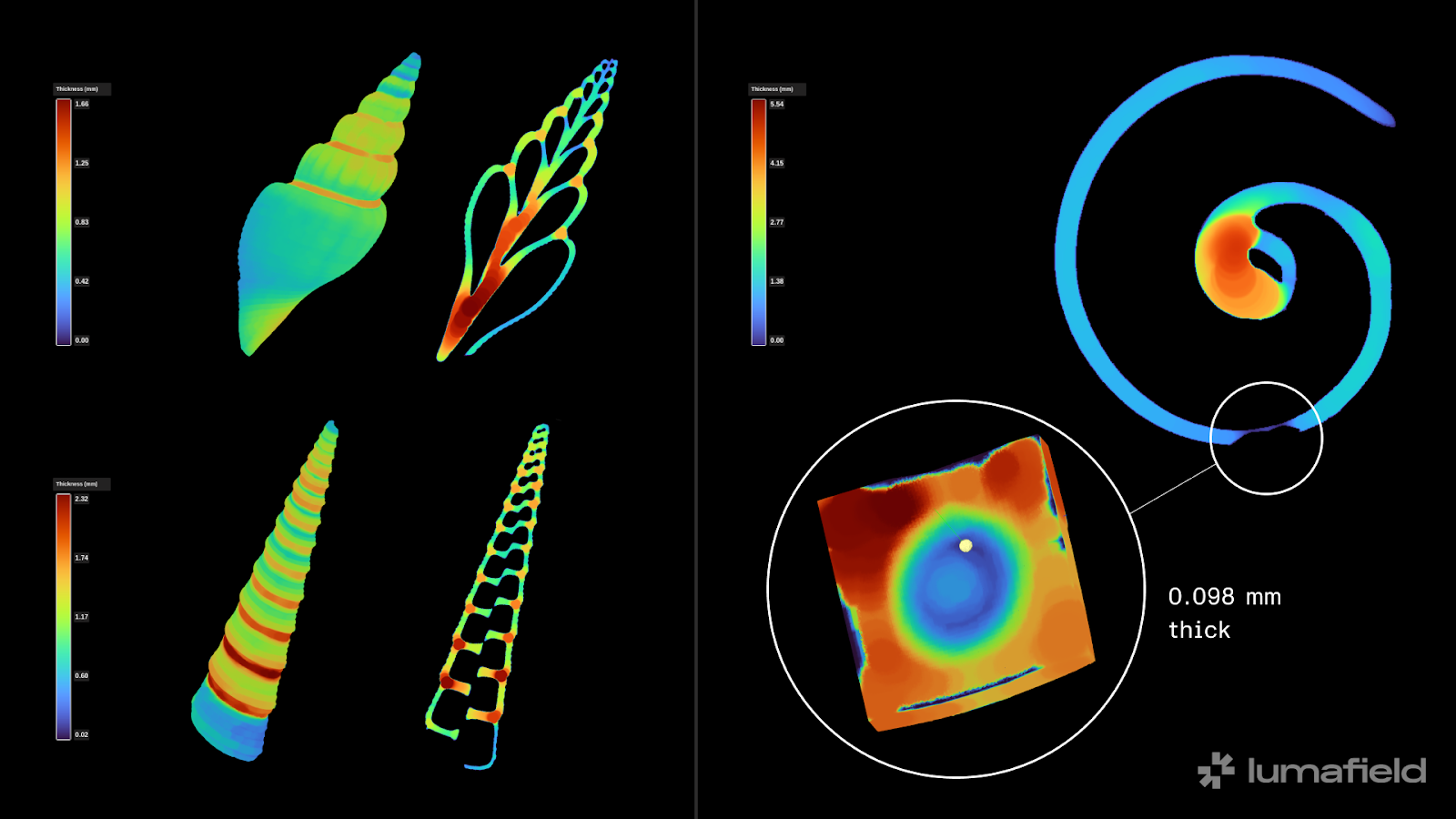
But the most revealing detail comes from a different specimen: the moon snail. Using the probe tool, we identified a shallow circular depression near the apex—likely the start of an octopus attack. Predatory octopuses drill through shells to reach the snail inside, and this kind of pit is consistent with that behavior. At its thinnest point, the indent measures only 0.098 mm. Without CT, this would be almost impossible to measure.
The Form of Function
Each of these shells solves the same problem differently. Some minimize material. Others proliferate complexity. Some yield perfect symmetry, others adapt to what life throws at them. What unites them is that these strategies were invisible—until now. CT scanning reveals the connection between form and function. It gives engineers and biologists alike a new way to study natural structure nondestructively, in 3D, and with the resolution needed to apply natural principles to human design. These aren’t just shells. They’re design histories, built from the inside out.